212 Astronomy Building
New Mexico State University
Las Cruces, NM 88003
Email: carrera4@nmsu.edu
Research: Formation and evolution of exoplanet systems
I am an Assistant Research Professor at the Department of Astronomy at New Mexico State University. I use computer models to study the formation and evolution of of planetary systems, from sticking collisions of small dust particles, to the formation of asteroids and the planet-building-blocks we call planetesimals, to the late-stage dynamical evolution of a fully formed planetary system.
I am the recipient and Principal Investigator of two NASA research grants to study the formation of the solar system and exoplanets:
Exoplanets Research Grant
There is growing evidence that planet formation begins earlier than previously thought. Planets form in a disk of gas and dust around a young star. Evidence from meteorites on Earth as well as ALMA observations that planet formation begins in the first 1 Myr, at a time when the disk is massive and its behavior is largely affected by its own self-gravity and by continuous in-fall of gas from the surrounding environment. This is in contrast with the more passive disks where most planet formation research has taken place.
I received a grant to study how the key stages of planet formation might be different in a young (< 1 Myr) disk. The stages include the growth of dust grains into cm-size pebbles, the formation of km-size planetesimals, and the growth of planetesimals into fully formed planets.Emerging Worlds Grant
I like to divide planet formation into three key stages: (1) Dust grains stick to become pebbles. (2) Pebbles come together to form km-size planetesimals. (3) Planetesimals have enough gravity to attract each other as well as pebbles, so they can grow into fully formed planets.
Of these, step (2) is arguably the least understood. Most proposals call for an aerodynamic process to concentrate pebbles into a dense enough region to undergo mutual self-gravity. Of these, the most popular idea is a process called the Streaming Instability (SI). A common analogy for the SI is that of cyclists coming together into a peloton in order to reduce air drag. Of course, cyclists do that intentionally and pebbles don’t have brains, but it is useful to think of a grouping of particles as a low-energy state, which is usually preferred by nature.
Aside from my current grants, I have conducted research on the Photo-evaporation of protoplanetary disks, on the growth of Super-Earths (planetary bodies larger than Earth, smaller than Neptune), Planet migration through the protoplanetary disk, and the dynamical stability of planetary systems.
MOVies
Explanations for the movies are shown after the carousel:

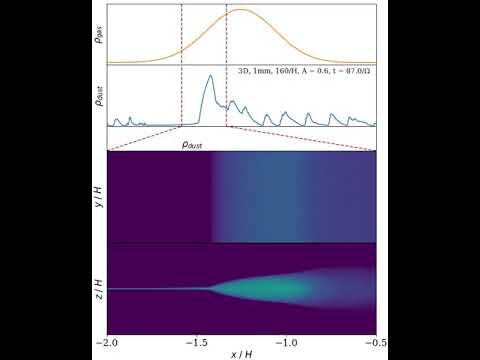
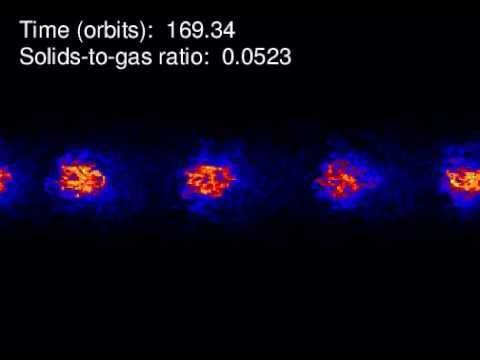
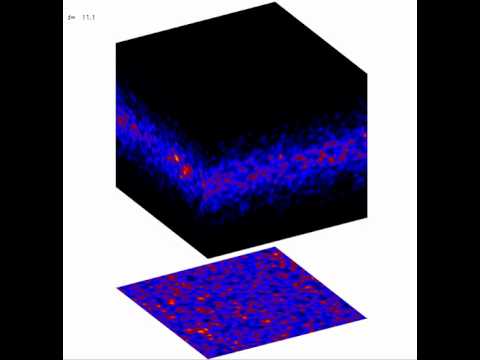
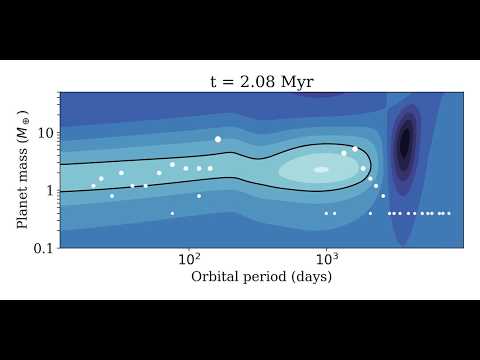
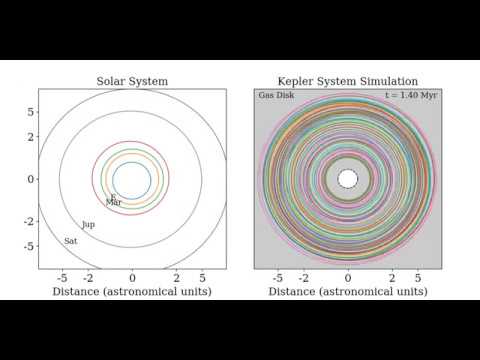
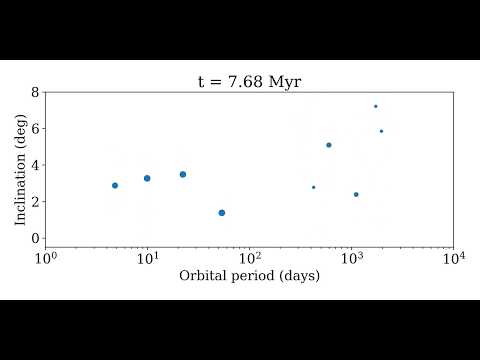
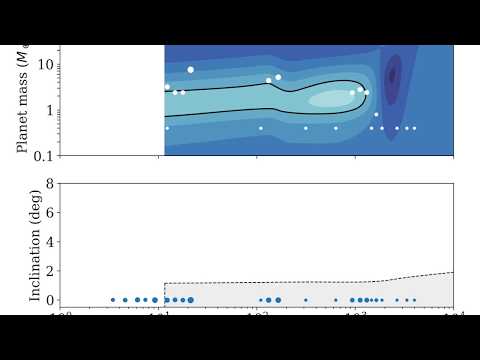
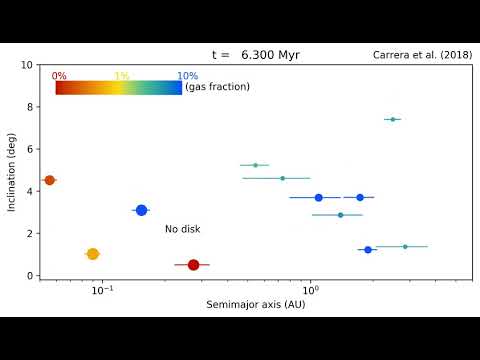
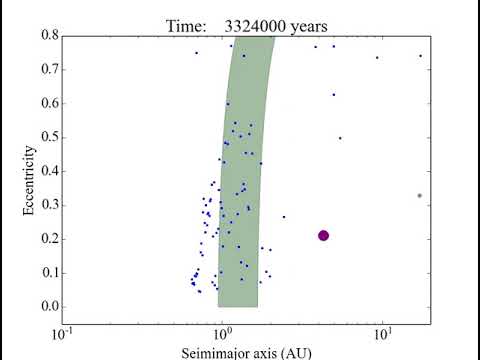
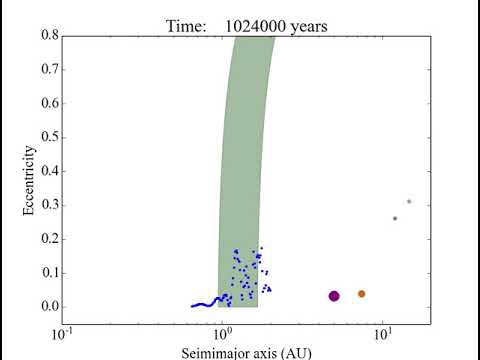
Movie explanations
Planetesimal formation in pressure bumps
The first two movies show the evolution of dust grains near a gas pressure bump in a protoplanetary disk. This might be the process that forms planetesimals (1-100 km rocks that are the building blocks of planets).
The first movie shows relatively large cm-size pebbles in a modest-sized pressure bump. As the particles pass through the pressure bump, they slow down slightly. This causes a local concentration of solids, similar to a traffic jam. The increase in density is high enough to trigger planetesimal formation by the streaming instability.
The second movie shows much smaller mm-size dust grains in a much larger pressure bump. This pressure bump forms a full particle trap. As particles enter the pressure bump, they become trapped. The particle density increases steadily until it triggers gravitational collapse, and a large particle clump forms without help from the streaming instability.
This work is part of Carrera et al. (2021) and Carrera & Simon (2022). See also Carrera et al. (2022).Planetesimals formation by the streaming instability
The next two movies show a close-up of how the streaming instability works to form planetesimals. These simulations consist of a small box inside a protoplanetary disk. The particle-gas drag leads the solids into dense filaments. These dense filaments may be the birthplace of planetesimals. In this investigation I ran a wide range of simulations with different particle sizes and densities, and I measured the density needed to trigger the streaming instability (and form dense filaments) as a function of particle size.
This work is presented in Carrera et al. (2015).Formation of super-Earths
The next six movies show the formation of a Kepler-like system with multiple super-Earths with short orbital periods. The simulations begin with 125 planetary embryos that first experience strong gravitational interactions and collisions. After about 1 Myr, the embryos merge into a smaller number of planets which settle into the midplane of the disk due to planet-disk interactions. Planets migrate and become locked into chains of mean motion resonances. Most of these chains would be dynamically unstable were it not for the effect of the disk dampening the orbital eccentricities. When the inner chain reaches the edge of the disk, the torque on the planets farther out force the inner planets to push past the disk edge, leading to the formation of planets with very short orbital periods.
This work is presented in Carrera et al. (2019a) and Carrera et al. (2018b).Survival of habitable planets in unstable planetary systems
The next two movies show the possible fate of a rocky planet in the habitable zone of a planetary system where planets become dynamically unstable. The giant planets have the mass of Jupiter, Saturn, Uranus, and Neptune, but they have smaller orbits which enhance the effect of planet-planet gravitational interactions. The habitable zone is shown in green. The evolution of the system is chaotic; small changes in the initial conditions can lead to vastly different outcomes. In one simulations, the instability is benign and most habitable planets survive. In the other simulation, the giant planets experience a catastrophic instability that completely decimates the habitable zone.
This work is presented in Carrera et al. (2016).
Publications
Rea, D.G., Simon, J.B., Carrera, D., et al., “Magnetically Driven Turbulence in the Inner Regions of Protoplanetary Disks”, 2024, ApJ, 972, 128R
Lim, J., Simon, J.B., Li, R., Armitage, P.J., Carrera, D., et al., “Streaming Instability and Turbulence: Conditions for Planetesimal Formation”, 2024, ApJ, 969, 130L
Cañas, M.~H., Lyra, W., Carrera, D., et al., “A solution for the density dichotomy problem of Kuiper Belt objects with multi-species streaming instability and pebble accretion”, 2024, PSJ, 5, 55C
Zawadzki, B., Carrera, D., & Ford, E. B., “Migration traps as the root cause of the Kepler dichotomy”, 2022, ApJ, 937, 53Z
Carrera, D., Simon, J. B., “The Streaming Instability Cannot Form Planetesimals from Millimeter-size Grains in Pressure Bumps”, 2022, ApJL, 933, 10
Auddy, S., Dey, R., Lin, M-K., Carrera, D., Simon, J. B., “Using Bayesian Deep Learning to infer Planet Mass from Gaps in Protoplanetary Disks”, 2022, ApJ, 936, 93A
Carrera, D., Thomas, A. J., Simon, J. B., Small, M. A., Kretke, K. A., Klahr, H., “Resilience of Planetesimal Formation in Weakly-Reinforced Pressure Bumps”, 2022, ApJ, 927, 52
Carrera, D., Simon, J. B., Li, R., Kretke, K. A., Klahr, H., “Protoplanetary Disk Rings as Sites for Planetesimal Formation”, 2021, AJ, 161, 96
Zawadzki, B., Carrera, D., & Ford, E. B., “Rapid formation of super-Earths around low-mass stars”, 2021, MNRAS, 503, 1390
He, M. Y., Ford, E. B., Ragozzine, D., & Carrera, D., “Architectures of Exoplanetary Systems. III. Eccentricity and Mutual Inclination Distributions of AMD-stable Planetary Systems”, 2020, AJ, 160, 6
Carrera, D., Ford, E. B., & Izidoro, A., “Formation of short-period planets by disc migration”, 2019, MNRAS, 486, 3874
Carrera, D., Ford, E. B., Izidoro, A., Jontof-Hutter, D., Raymond, S. N., & Wolfgang, A., “Identifying inflated super-Earths and photo-evaporated cores”, 2018, ApJ, 866, 2
Carrera, D., Davies, M.B., Johansen, A., “Toward an initial mass function for giant planets”, 2018, MNRAS, 478, 961.
Yang, C.-C., Johansen, A., Carrera, D., “Concentrating small particles in protoplanetary disks through the streaming instability”, 2017, A&A, 606, A80.
Carrera, D., Gorti, U., Johansen, A., Davies, M.B., “Planetesimal Formation by the Streaming Instability in a Photoevaporating Disk”, 2017, ApJ, 439, 16.
Carrera, D., Davies, M.B., Johansen, A., “Survival of habitable planets in unstable planetary systems”, 2016, MNRAS, 463, 3226.
Carrera, D., Johansen, A., Davies, M.B., “How to form planetesimals from mm-sized chondrules and chondrule aggregates”, 2015, A&A, 579, 43.
Education
Ph.D., Astronomy & Astrophysics, Lund University, 2017.
Thesis: Formation and Early Evolution of Planetary Systems.
M.S., Astronomy & Astrophysics, Lund University, 2012. Graduated with Distinction.
Thesis: The effect of dark matter capture on binary stars.
B.Sc., Math & Physics, University of Toronto, 2001. Graduated with Distinction.